treatment for obesity in animals
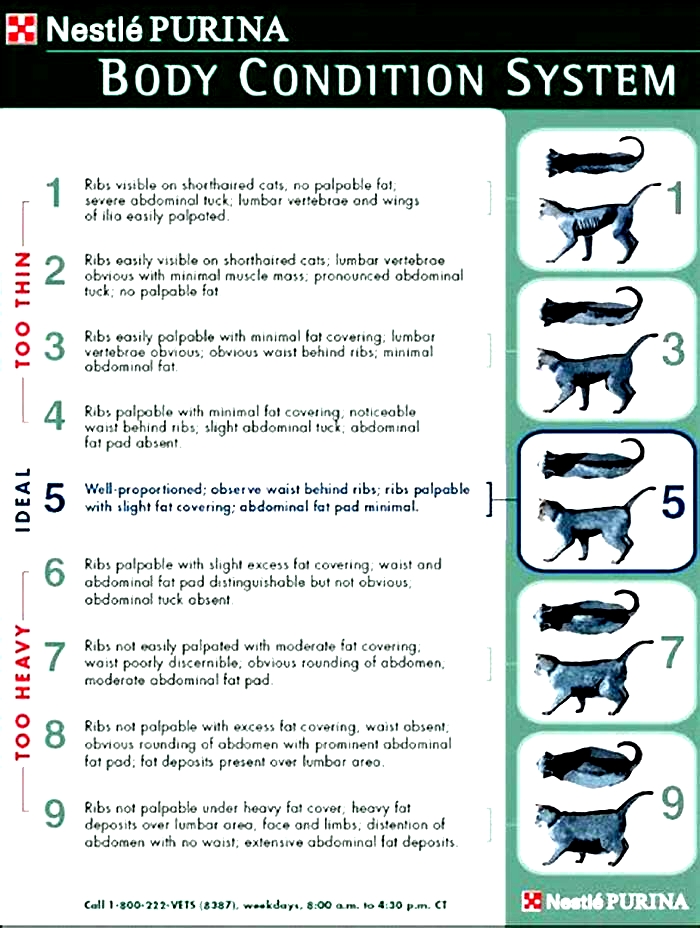
Treatment of Obesity in Cats and Dogs
To provide the best experiences, we use technologies like cookies to store and/or access device information. Consenting to these technologies will allow us to process data such as browsing behavior or unique IDs on this site. Not consenting or withdrawing consent, may adversely affect certain features and functions.
The technical storage or access is strictly necessary for the legitimate purpose of enabling the use of a specific service explicitly requested by the subscriber or user, or for the sole purpose of carrying out the transmission of a communication over an electronic communications network.
The technical storage or access is necessary for the legitimate purpose of storing preferences that are not requested by the subscriber or user.
The technical storage or access that is used exclusively for statistical purposes.The technical storage or access that is used exclusively for anonymous statistical purposes. Without a subpoena, voluntary compliance on the part of your Internet Service Provider, or additional records from a third party, information stored or retrieved for this purpose alone cannot usually be used to identify you.
The technical storage or access is required to create user profiles to send advertising, or to track the user on a website or across several websites for similar marketing purposes.
A systematic review on different models of inducing obesity in animals: Advantages and limitations
Introduction
Obesity is one of the worrisome health issues in advanced societies; it is a complex disorder that involves an excessive amount of body fat [1,2]. Obesity is characterized by an increased body mass index (BMI) of 30 kg/m2 and above [3,4]. It is not just a cosmetic concern but is associated with other health diseases, such as hypertension, coronary heart disease, and diabetes [5]. Besides, it also associates with respiratory and prolonged musculoskeletal problems, lumbago, subfertility, and skin disease. For the past 30 years, its prevalence has increased tremendously [6], especially in the developing countries leading to the manifestation of obesity and its comorbidities like diabetes, which stimulated the mixture of the two words as diabesity. Hence, to battle obesity, the improvement of non-toxic and effective therapeutics is required [7]. Over the years, the use of animal models remains indispensable for finding, authenticating, and making effective original therapeutics for their harmless use in humans.
Similarly, it brings a desirable transition from the research done in the laboratory to new ways of treating patients. Consequently, the advantage of using animals models is that they can be kept up in strictly controlled conditions, fed with standard diet regimen, and kept under conditions without pathogens or germs. However, the limitation of animal models is that there is no affirmation that conditions which are externally comparable in animals and man are comparable at their fundamental level. In recent times, researchers have been faced with problems of selecting suitable animal models, thus having a very great number of contradicting factors and drawing inappropriate conclusions. Distinctive animal obesity models, ranging from monogenic models to surgical models are significant in examining the conceivable etiology, pathogenesis, and treatment of the obesity condition in people, gave both the favorable advantages and limitations are completely comprehended [8]. This review thus aims at analyzing the various animal models of inducing obesity, their advantages, and limitations. Information on keywords were gotten from EBSCOHOST, Google Scholar, Science Direct, SCOPUS, Springerlink, and PubMed databases from 2000 to 2019 based upon which the animal models were reviewed.
Classification of obesity
Excessive aggregation of fat in the body causes obesity bringing about negative impacts on the health of the individual [9]. Waist Circumference (WC), WaistHip Ratio (WHR), and BMI are the components utilized in the analysis of obesity. WHR is a potential marker of other increasingly severe health conditions. According to the World Health Organization, abdominal obesity is characterized as a WHR of more than 0.85 and 0.90 for females and males, respectively [10].
Similarly, females with WHR of more than 0.8 and males with more than 1.0 are at a higher risk for health problems. WHR has been demonstrated to be a superior indicator of cardiovascular illness than WC and BMI. WHR, which shows the central body fat distribution, is recently proved to be a marker for the risk of health problems. WHR can be calculated by dividing WC with the hip circumference.
Based on the clinical guideline for the identification, assessment, and management of obesity, adult patients are classified according to their co-morbidity risk status. Classification of Diseases Ninth Revision (ICD-9) is utilized to recognize persistent co-morbidities [11]. In Organization for Economic Co-operation and Development (OECD) countries, about half of the adults are having BMI 25 Kg/m2 or overweight [12,13]. summarizes the classification of overweight, obesity, and WC. Different types of obesity classified based on their origin have been identified (). Overweight is due to taking more food than the individuals activity level, which can increase fat storage (exogenous) or due to dysfunction of metabolic or hormonal systems (endogenous).
Table 1.
Classification of overweight and obesity by BMI, WC and associated disease risk.
Disease risk * (relative to normal weight and waist circumference) | ||||
---|---|---|---|---|
BMI (kg/m2) | Obesity class | Men 40 in (102cm)Women 35 in (88cm) | >40 in (102cm)>35 in (88cm) | |
Underweight | < 18.5 | |||
Normal | 18.5 24.9 | |||
Overweight | 25.0 29.9 | Increased | High | |
Obesity | 30.0 34.9 | I | High | Very high |
35.0 39.9 | II | Very high | Very high | |
Extreme obesity | 40 | III | Extremely high | Extremely high |
Table 2.
Summary of types of obesity.
Types of obesity | Sex | Body region affected | Diseases associated with | References |
---|---|---|---|---|
Central/abdominal, android or apple | Male | Abdomen | Metabolic disorders | [14] |
Peripheral/visceral, gynaecoid or pear | Female | Buttocks, hips, and thighs | Infrequently associated with metabolic disorders | [1] |
Diffuse | Both | Whole body | - | [15] |
Localized | Both | Barraquer-Simons Syndrome, lipodystrophic disorders, | [16] | |
Formerly obese | Both | Skin (redundant cutaneous mantle) | - | [17] |
Childhood | Children, adolescent | Whole body | fatty liver disease, Type 2 diabetes, asthma, fatty liver disease, cardiovascular disease | [18] |
Morbid | Both | Whole body (BMI of more than 40) | high blood pressure or diabetes | [19] |
Sarcopenic | Both | Low muscle mass, muscle strength, and high fat | geriatric syndromes | [20] |
Mechanisms of several diseases in obesity
Increased adipocytes have detrimental consequences on the pancreas, liver, kidneys, brain, heart, reproductive organs, muscles, and joints. The synthesis of adipokines triggers the proinflammatory cytokines, which impairs insulin in the pancreas leading to inflammation and consequently, Type 2 diabetes becomes inevitable [21]. The excessive production of lipids accumulates in the liver tissues causing increased lipotoxicity, which results in fatty liver disease, steatohepatitis, and cirrhosis. Similarly, increased adipocytes lead to mechanical stress on the kidneys, muscles, and joints resulting in renal compression and mechanical load on the joints, respectively, which ultimately leads to kidney failure and osteoarthritis [22]. The increased insulin resistance affects the brain by increasing neuronal insulin which also triggers the leptin action to cause neuronal inflammation and ultimately leading to hippocampal neurodegeneration and memory impairment. The effect of increased adiposity on the heart results in fat accumulation on the myocardium. This process increases triglycerides hydrolysis to form free fatty acids and causes dyslipidemia. This, in turn, leads to coronary heart disease [23]. Furthermore, reproductive systems are not spared, as the accumulation of fats in reproductive organs causes the release of reactive oxygen species, resulting in decreased sexual behavior, performance, and fertility. The mechanism through which obesity can cause several diseases is summarized in .
Summary for the recommended hypotheses on the mechanisms through which obesity can cause several diseases.
Epigenetic considerations in obesity
Epigenetics is the alteration in a chromosome that damages the activity of a gene for which its expression can be inheritable by offspring. These alterations may include the addition of methyl, carboxyl, and hydroxyl groups to the DNA nucleotides. Recently, epigenetics suggests that obesity in males alters offsprings metabolic and reproductive phenotypes by re-adjusting of spermatogonial stem cells [24]. These effects are also called epigenetic effects and were first discovered in human models. However, animal models still can give a significant model to investigate the mechanisms because it is ethically wrong to impose restrictions on human fetuses, while small animals can make the timescale effect possible to study. In a study carried out by Fraga et al. [25], monozygotic twins are epigenetically identical for some time in their lives, but with time notable changes were visible in their DNA and histone acetylation. Such alterations induced by the environment may have effects on their BMI. Besides, in research done in the Netherlands, in utero and early childhood, exposure to starvation had higher adverse effects on weight and height during adulthood [26].
Models of inducing obesity in animals and their advantages and limitations
There are several models of producing obesity in animals, which can be classified as (1) Genetic and (2) Non-genetic. Genetic models include monogenic, polygenic, and transgenic models, while the non-genetic models consist of dietary, exotic, large animals, and surgical models ().
Schematic diagram showing the obesity models. Legend: 11beta HSD-1: 11beta-hydroxysteroid dehydrogenase type 1; AgRP: agouti-related peptide overexpression; ARC: Arcuate Nucleus; C3H: C3H/HeJ mice; CRF: corticotrophin releasing factor; db/db: diabetic mouse; DIO: diet-induced obese; DR: diet resistant; GLUT4: glucose transporters 4; HFD: high-fat diet; HS: high-sucrose; KK: Kuo Kondo; MC3R: melanocortin 3 receptor knockout in mice; MC4R: melanocortin 4 receptor knockout mice; MCH: melanin concentrating hormone; NPY: Neuropeptide-y; NZO: New Zealand Obesity; ob/ob: obesity mouse; OLETF: Otsua Long Evans Tokushima Fatty; POMC/AgRP: Pro-opiomelanocortin/agouti-related peptide knockout mice; POMC: Pro-opiomelanocortin knockout; PVN: Paraventricular Nucleus; s/s mouse; TSOD:Tsumura and Suzuki obesity and diabetes; VMH: Ventromedial Hypothalamus; WDF: Wistar Kyoto fatty; WFR: wistar fatty rat; WHR: Waist-to-Hip Ratio; ZDF: Zucker Diabetic Fatty; ZFR: zucker fatty rats; MSH: -melanocyte-stimulating hormone.
Monogenic model of obesity
The monogenic model provides a unique insight into the organic mechanisms that lead to obesity [27]. Monogenic obesity is due to a mutation(s) in the leptin-melanocortin pathway [28]; hence, a few investigations have established that a minimum of 10 single gene impairments can cause obesity and single gene impairment can also result in dysregulation in different modes of energy expenditure [29]. Mutations that occur at the leptin and its receptors are typically found in obesity (ob/ob) mouse [30,31], diabetic (db/db) mouse [32], s/s mouse [33], Zucker (fa/fa) [34], and Koletsky obese rats [35], other monogenic models that have downstream deficits on the leptin receptor are, Wistar Kyoto fatty rats [36], POMC knockout [37,38], POMC/agouti-related peptide (POMC/AgRP) knockout mice [39], melanocortin 4 receptor (MC4R) knockout mice [40], melanocortin 3 receptor (MC3R) knockout [41] in mice, agouti-related peptide (AgRP) overexpression [42,43] (). The ob/ob mouse model provides the molecular basis for obesity study; the obese gene was identified in 1949 in the Jackson Laboratory by researchers who discovered it accidentally [44]. The monogenic model is the most used. The studies have revealed that mice can attain a weight three times more than unaffected mice. It was found that the obese mice had enlargement of the pancreas and increased production of insulin, leading to hypercorticosteronemia, insulin resistance, hyperglycemia, hyperinsulinemia, and hypothyroidism as well as infertility [45].
Consequently, db/db mouse model also provides the molecular basis for obesity study. It was discovered in 1966 at the Jackson Laboratory, and the model has been used for over 50 years. In the gene of leptin receptor of these mice, the mutation occurs at G-to-T point, which leads to diabetes, dyslipidemia, high leptin, and insulin levels and insulin resistance. Besides, at the age of 8 weeks, they develop hyperglycemia. They are commonly used as type 2 diabetes animal model [46].
In s/s mouse model, there is a mutation that aims to disturb a transcription factor named STAT3, a fundamental component for the long-form signaling pathway of the leptin receptor [47,48]. They create inflexible insulin resistance in the liver yet are less hyperglycemic. Similarly, mutation of the leptin receptor (fa/fa) occurs in Zucker and Zucker Diabetic Fatty rats. They build up a phenotype of hyperphagia, while Koletsky rats change leptin receptor (null-transformation) [37]. Then, in 1981, Wistar fatty rat (WFR) was reported by [49] in which it was obtained by transferring fa gene from Zucker fatty rats with 13 M strain to Wistar Kyoto rats that had impaired glucose tolerance. WFR is obese early in its life and has diseases related to obesity, like type 2 diabetes, hyperlipidemia, and hyperinsulinemia.
POMC, located in the hypothalamic arcuate nucleus, is a precursor of alpha-melanocyte-stimulating hormone (MSH), which is the target of leptin and its absence usually leads to obesity [50]. Similarly, the mice with POMC/AgRP knockout often possess double knockout for AgRP and POMC. They develop obesity during increased eating because of AgRP acting on the MC4 receptor. The MC4R knockout mice are created to target the MC4 receptor, thereby producing hyperphagia and morbid obesity [51,52], while the MC3R mice become obese when MC3 receptor is inactivated leading to increase fat accumulation [53]. Then again, MC4/MC3 receptor knockout mouse is those having double knockout on the MC3 and MC4 receptors. The obesity gene was taken to another level in 1992 with the discovery of ectopic agouti expression mice, which showed the cloning of the agouti gene.
Similarly, the AgRP expression mouse is the homologous of agouti receptor and a natural antagonist of MSH at MC3 and MC4 receptor [54]. The monogenic model has proven to be the most reliable animal obesity models, until now it remains the most effective models. It is very cost effective and easy to maintain. It has also shown the ability to make a large population during mating; this is obvious in db/db and ob/ob mice. The model differs from human obesity in terms of energy partitioning and fat deposition and does not represent the obesity in human. It is very difficult to carry out; therefore, it requires a technical knowhow [5557].
Polygenic model of obesity
Inheritance of a quantitative phenotype is regulated and altered by a set of alleles at the different gene loci called polygenic variants, which also modifies the expression of a qualitative character. These variants vary in different individuals and play a role in body weight regulation [58]. Obesity develops when many polygenic variants in an individual increase body weight. It is worthy to note here that each polygene only contributes a fraction to the build-up of obesity [59]. A couple of these variants are found in obese individuals, as well as in normal-weight and lean individuals at a low level. The several types of polygenic models include New Zealand Obesity (NZO) mouse [60], Tsumura and Suzuki obesity and diabetes (TSOD) [61], C3H/HeJ mice (C3H) [62], Kuo Kondo (KK) [42], M16 [63], PBB/Ld [64], BRSUNT/N [56], 7L/IRE [56], Otsua Long Evans Tokushima Fatty (OLETF) [65], Sand rats, spiny mouse [66], and Tuco-tuco rats [67] (). The NZO group of mice displays obesity due to increased body weight within the initial 2 months, which may be due to hyperphagia related to leptin resistance even with hereditarily normal leptin and leptin receptors [68].
NZO mice have the severe phenotype, fat deposits, and diminished exercise activity relative to ob/ob and control mice [69]. Hence, obesity in NZO mice is because of hyperphagia, lack of physical activity, and diminished energy expenditure, which makes it similar to human obesity [42]. TSOD includes TSOD strain, which is obese with diabetes and Tsumara Suzuki non-obese strain, which is not obese [70]. M16 mouse model is an outbred model for the early development of polygenic obesity. It is created via the long-term selection of weight gain for 3 to 6 weeks. As compared to controls, it shows hyperphagia, hyperinsulinemia, and hyperleptinemia. At eight weeks of age, compared to controls, the females and males of this strain are hyperglycemic, in which they had 22% and 56% higher fasting plasma glucose levels, respectively [42].
KK Mouse is likewise a polygenic obesity model that shows insulin resistance, hyperinsulinemia, and hyperphagia with a moderate obesity at eight weeks of age. It was created with certain inbreeding for huge body size in Japan. The lethal yellow obese gene (Ay) was transferred to KK mouse strain to develop another strain named KKAy mouse which is broadly utilized for obesity and diabetes studies [42]. Another strain of rats for obesity models known as OLETF is created in Japan. Several weeks after delivery, these rats are typically hyperphagic with higher body weight leading to obesity. These rats are commonly utilized in diabetes and obesity studies. Obesity and diabetes-like disorder are reported in laboratory animal species like Israeli sand rat (Psmmon:ys obesus), the spiny mice (Acomys cahirimus, A. russatus), the tuco-tuco (Etenomys taerum), and Djungarian hamster (Phodopus sungorus). Polygenic obesity remains the most frequently used animal model of obesity; it is cost-effective and does not require prior knowledge. Although this model remains a more realistic model of human obesity, it is time-consuming, lacks quality standardization, and the size of mice causes severe limitations of this model [42,59,71].
Transgenic model of obesity
To understand the mechanism, transgenic models of obesity have been created. These include corticotrophin-releasing factor (CRF) overexpressing mice [72], animals with enhanced GLUT4 glucose transporters [73], mice with overexpression of serotonin 5-HT-2c [74], melanin-concentrating hormone (MCH) [75], beta-3 adrenergic [76], neuropeptide-Y (NPY) 1 [77]/NPY2 [78]/bombesin 3/neuronal insulin receptor knockout (NIRKO) mice [79], and mice with overexpression of 11beta-hydroxysteroid dehydrogenase type 1 (11beta HSD-1) [80] (). CRF transgenic mice show central obesity with comorbidities, such as hair loss, thin skin, and muscle wasting. CRF is produced by the paraventricular nucleus (PVN) in the hypothalamus and is the main component of the hypothalamic-pituitary-adrenal axis [81].
Obesity usually develops in transgenic mice expressing GLUT4 in adipocytes by increasing nutrient substrate for adipogenesis and insulin-stimulated glucose transport. The number of adipocytes, not the size, is increased. Hence, they are used as models of fat-cell replication and differentiation during obesity development [82]. The transgenic mice overexpressing MCH develop obesity late in life. They are also associated with insulin resistance, hyperphagia, and high insulin level [83]. Similarly, mice with serotonin receptor (5-HT-2c) knockout that have a smaller number of functional 5-HT2C receptors develop hyperphagia [84,85] in which adiposity and body weight are increased during weaning. Meanwhile, mice with knockout of neuropeptide-Y 1 receptor (NPY1R) have hyperphagia due to low energy expenditure accompanied by low uncoupling protein type-2 (UCP2) expression in white adipose tissue leading to obesity [77].
Furthermore, mice with NPY2R knockout develop paradoxical obesity [86]. They become mildly hyperphagic and develop obesity. Mice with knockout of Bombesin 3 receptor (BRS3 ko) are hemizygous for this receptor, and they establish late-onset obesity because of hyperphagia and decreased metabolic rate [87]. They also have hyperglycemia, insulin resistance, and a high level of insulin. NIRKO mice take moderate food, resulting in increased body weight, adiposity, hypertriglyceridemia, and high insulin level [88]. The aftermath is conspicuous in mice exposed to high-fat diet (HFD), but female NIRKO mice develop obesity when on low chow. Mice with 11beta HSD-1 overexpression specifically in fat tissue have increased corticosterone level in fatty tissue, visceral obesity, and most metabolic syndrome features [89]. As compared to wild-type controls, fat-specific 11beta HSD-1 transgenic mice consume food more, in which they have insulin resistance and the likelihood to develop diabetes. The transgenic model, on the other hand, has hereditary etiology, very reliable, and effective in the induction of obesity. One of its significant advantages is that it is targeted at a particular gene. There are so many genetic tools available for its processes, but unfortunately, it requires an in-depth knowledge of its procedures [36,9092]
Diet-induced model of obesity
Diet-induced obese (DIO) rats develop obesity when given HFD, while diet-resistant rats have body weights like control rats when fed with a low energy diet. At 4 to 5 weeks of age, during they are lean and before their body weight starts to diverge, DIO rats become less sensitive to the hypophagic activity of leptin [93]. It is evident that animals introduced to HFD usually develop obesity and can exhibit reduce levels of insulin and leptin sensitivity [94]. Cafeteria diets are apparent imitations of models of human obesogenic foods. They result from excessive eating that is made up of increases in the energy expenditure, a result of sympathetic activation of brown adipocyte. Overconsumption of cafeteria diets means there are an increase in the frequency and average meal size.
These diets provide animals with a mixture of sugar, salt, and high fat drawn from solid foods [95,96]. Additionally, in the high sucrose (HS) DIO rat model, the rats are fed with this diet or a modified HS diet for a few weeks. Visceral adipose tissue is enlarged by the exposure of rats to HS diet without necessarily increasing body weight and reducing glucose disposal rates. An increase in hepatic glucose, plasma glucose, and free fatty acids are all associated with this model. Lipidosis and swelling of hepatocyte mitochondria in the liver are found in HS rats [97]. The advantages of the diet-induced model of obesity include having a combination of genetic and dietary influences on the animal; it can also be a quick way of inducing obesity and is insulin resistant. There is a substantial similarity to human obesity, and the model is cost-effective. The limitations of the model are poor standardization, long duration, and they are overtly obese.
Exotic model of obesity
The model comprises wild animals that go through original designs of disparity in their fat mass, such as seals and bats [90]. However, the benefit of this model is influenced by the absence of instruments to investigate a genetic basis, and the animals present a huge challenge with regards to setting up research facility-based colonies for the experiments. The model is known for its efficacy and will likely give unique insights about the storage of body fat [71]. Exotic models are non-human primates and non-standard small rodents that experience periodically induced fat storage and exhibit photoperiods. The main limitation of this model is its inability to establish laboratory colonies, and the tools for exploring its genetic basis have not been developed.
Non-human primate model of obesity
The non- human primate model exhibits obesity, which is similar to human obesity. It involves the old worlds monkeys, for example, macaques, rhesus monkeys, and baboons, which give appropriate and essential information related to human obesity [90,92]. Rhesus monkeys raised in cages tend to have increased body weights and develop obesity with its comorbidities [42]. Furthermore, when on food ad libitum, obesity is visible in macaques in an age-dependent manner. They also develop type 2 diabetes, as well as its complications. Their body inactivity increases the risk of obesity. Spontaneous obesity is also detected in wild baboons and free-ranging rhesus monkeys. Japanese monkey named Macaca fuscata also becomes obese without diabetes [42]. The non-human primate model is fundamentally the same as the human model of obesity, and this is a direct result of its closeness in structure (anatomy) and function (physiology). The capacity to conduct blood sampling endoscopy and laparoscopic biopsies make the model very unique. However, its high cost of maintenance, lack of approved facilities, long life cycle, and uniparity have limited the use of this model.
Seasonal model of obesity
The seasonal model of obesity is closely related to human obesity in several ways. For instance, high fat-fed hamsters have similar findings in humans about the distribution of phospholipid classes, the activity of cholesterol ester transfer protein, and regulation of LDL receptor [98]. They are, therefore, suitable for the investigation of dyslipidemia and cholesterol metabolism. Diets consisting of 12%15% of fat and 0.3%0.5% of cholesterol induces dyslipidemia, hyperglycemia, and moderate obesity in hamsters. There are two kinds of hamster strains which can be used for the induction of dyslipidemia, namely, BioF1B hamsters and Lakeview Golden (LVG) outbred Lakeview hamsters (Charles River) [46].
Large animals model of obesity
Larger animals model of obesity has a lot of resemblance to human obesity because it allows a broad metabolic phenotyping assessment of obesity. The examples of such large animals include dogs, pigs, and cats [42]. As compared to humans, domestic dogs may have an epidemic of obesity, which can be more extreme. HFD-induced obesity in dogs is characterized by insulin resistance, high insulin level, and impaired glucose tolerance. Obese cats develop type 2 diabetes with the presence of -cell mass loss and islet amyloid [99]. On the other hand, dogs can have obesity within 412 weeks when they are free to access to (1) standard meat and chow diet, (2) meat and chow diet added with fat, or (3) commercial diet mixed with either high-fructose or high-fat or both ad libitum. Hyperphagia is maintained throughout the access, but higher energy intake is significant during the first 12 weeks [90].
The large animal model is the only animal obesity model that truly represents the human obesity. Their pharmacokinetics is similar to humans, and there are also available genetic tools for the model. In this model, canulation is possible; however, the model is very complex and complicated as specialized equipment and facilities are required to carry out the processes. Besides, the animals have a long-life cycle and not well characterized. Likewise, the non-mammalian model has a meager cost of maintenance when compared to other models of obesity, it has a short life cycle, and however, its major limitation is its distinct anatomy and physiology which makes it challenging to study or used as an extrapolation with human to obesity.
Surgical model of obesity
The damage to arcuate nucleus (ARC), PVN, ventromedial hypothalamus (VMH), and ovariectomy are examples of surgical models of obesity. Electric current and monosodium glutamate (MSG) causes bilateral lesions of the hypothalamic nuclei, which results in hyperphagia, adiposity, and increased body weight. This model requires anesthesia and surgical skills. The rats are anesthetized and bilateral VMH lesions produced by electrical destruction using stereotaxic instrument [100]. Obesity can also be induced by the extensive lesions of the PVN, which eventually may result in hyperinsulinemia and insulin resistance in rats [101]. It is worthy to note; it is hard to perform selective surgical lesions of the ARC because of its location and anatomical shape. So far, most lesions are involved the whole mediobasal hypothalamus, involving the ventromedial area. As another option, induction of relatively selective damage of ARC neurons projecting to PVN and VMH can be done by giving MSG repeatedly to neonatal rats ten days after delivery. Obesity develops in these rats as they become hyperphagia and have hyperinsulinemia and insulin resistance [102].
Studies carried out in rats suggest that there is an abrupt hormone deprivation caused by oophorectomy (surgical removal of the ovaries) [103]. The reduction of hormone level such as estrogen level leads to obesity and its metabolic sequelae. The surgical removal of ovaries reduces the initial leptin levels and increases the same after 7 weeks. This resistance to leptin may increase the weight gain of the rats. Animal studies show a consistent correlation between bilateral oophorectomy and adiposity, total and LDL-cholesterol levels, and insulin resistance. The surgical model is very reliable and cost-effective. Its primary advantage is that the effect of cytotoxic chemicals on other organs of the body can be avoided [100]. However, the limitations of this surgical model outweigh its advantages as, for instance, the VMH, PVN, and ARC nucleus are very difficult to locate, and the procedure requires high technical knowledge and post-operative processes, and it has high mortality rate [91].
The induction of obesity in different animal models is influenced by a few factors, including biological, psychosocial, and environmental factors. Hence, it is justifiable that various limitations are established when analyzing results obtained from different obesity models in a laboratory and humans. Although animal models are a significant technique for examining the impacts of obesity and drug testing, it is essential to comprehend the limits of the models overall capacity to mimic the pathophysiology of obesity in humans. The advantages and limitations of animal models of obesity based on technical know-how, duration, life cycle, cost, effectiveness, shape, mortality rate, characterization, complexity, approved facilities, ability to form colonies, time consumption, standardization, and ability to represent human diseases are summarized in .
Table 3.
Advantages and limitations of animal obesity models.
S/n | Type of Model | Type of Animal | Ecological classification | Advantages | Limitations |
---|---|---|---|---|---|
1. | Monogenic | Mice and rats | Genetic |
|
|
2. | Polygenic | Mice | Genetic |
| |
3. | Transgenic | Mice | Genetic |
| |
4. | Diet-induced | Mice and rats | Nutritional |
| |
5. | Exotic | Seal and bats | Nutritional | ||
6. | Non-human primates | Macaques, rhesus monkey and baboons | Nutritional | ||
7. | Seasonal | Hamsters | Environmental | ||
8. | Non-mammalian | Fish except zebra fish | Nutritional |
| |
9. | Large animals | Dogs, pigs and cats | Nutritional |
| |
10. | Surgical | Rats | Neural and endocrine |
|